Towards standards for human fecal sample processing in metagenomic studies
Technical variation in metagenomic analysis must be minimized to confidently assess the contributions of microbiota to human health. Here we tested 21 representative DNA extraction protocols on the same fecal samples and quantified differences in observed microbial community composition. We compared them with differences due to library preparation and sample storage, which we contrasted with observed biological variation within the same specimen or within an individual over time. We found that DNA extraction had the largest effect on the outcome of metagenomic analysis. To rank DNA extraction protocols, we considered resulting DNA quantity and quality, and we ascertained biases in estimates of community diversity and the ratio between Gram-positive and Gram-negative bacteria. We recommend a standardized DNA extraction method for human fecal samples, for which transferability across labs was established and which was further benchmarked using a mock community of known composition. Its adoption will improve comparability of human gut microbiome studies and facilitate meta-analyses.
This is a preview of subscription content, access via your institution
Access options
Access Nature and 54 other Nature Portfolio journals
Get Nature+, our best-value online-access subscription
cancel any time
Subscribe to this journal
Receive 12 print issues and online access
206,07 € per year
only 17,17 € per issue
Buy this article
- Purchase on SpringerLink
- Instant access to full article PDF
Prices may be subject to local taxes which are calculated during checkout
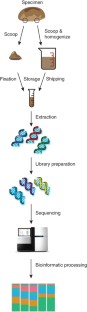
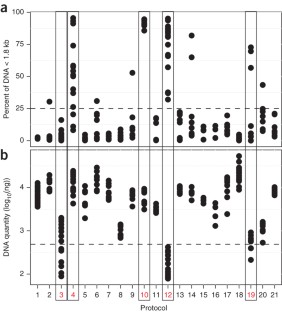
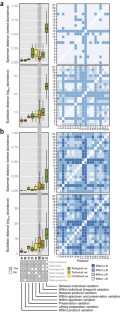
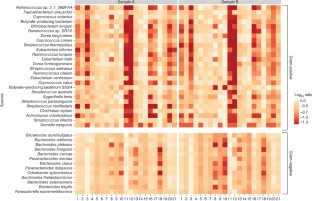
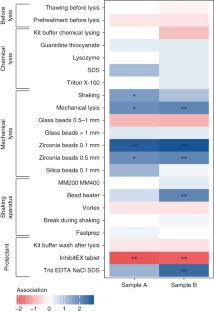
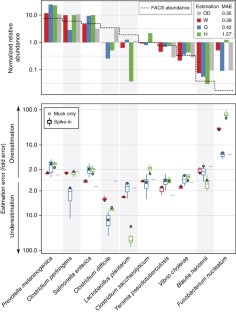
Similar content being viewed by others
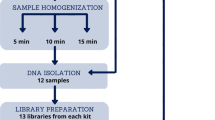
The standardisation of the approach to metagenomic human gut analysis: from sample collection to microbiome profiling
Article Open access 19 May 2022
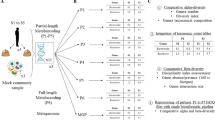
Multicenter evaluation of gut microbiome profiling by next-generation sequencing reveals major biases in partial-length metabarcoding approach
Article Open access 18 December 2023
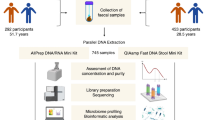
Choice of DNA extraction method affects stool microbiome recovery and subsequent phenotypic association analyses
Article Open access 16 February 2024
Accession codes
Primary accessions
European Nucleotide Archive
References
- Meyer, F. et al. The metagenomics RAST server - a public resource for the automatic phylogenetic and functional analysis of metagenomes. BMC Bioinformatics9, 386 (2008). ArticleCASPubMedPubMed CentralGoogle Scholar
- Larsen, N. et al. Gut microbiota in human adults with type 2 diabetes differs from non-diabetic adults. PLoS One5, e9085 (2010). ArticlePubMedPubMed CentralGoogle Scholar
- Qin, J. et al. A metagenome-wide association study of gut microbiota in type 2 diabetes. Nature490, 55–60 (2012). ArticleCASPubMedGoogle Scholar
- Forslund, K. et al. Disentangling type 2 diabetes and metformin treatment signatures in the human gut microbiota. Nature528, 262–266 (2015). ArticleCASPubMedPubMed CentralGoogle Scholar
- Manichanh, C. et al. Reduced diversity of faecal microbiota in Crohn's disease revealed by a metagenomic approach. Gut55, 205–211 (2006). ArticleCASPubMedPubMed CentralGoogle Scholar
- Carroll, I.M. et al. Molecular analysis of the luminal- and mucosal-associated intestinal microbiota in diarrhea-predominant irritable bowel syndrome. Am. J. Physiol. Gastrointest. Liver Physiol.301, G799–G807 (2011). ArticleCASPubMedPubMed CentralGoogle Scholar
- Zeller, G. et al. Potential of fecal microbiota for early-stage detection of colorectal cancer. Mol. Syst. Biol.10, 766 (2014). ArticlePubMedPubMed CentralGoogle Scholar
- Dethlefsen, L., McFall-Ngai, M. & Relman, D.A. An ecological and evolutionary perspective on human-microbe mutualism and disease. Nature449, 811–818 (2007). ArticleCASPubMedGoogle Scholar
- Dominguez-Bello, M.G. et al. Delivery mode shapes the acquisition and structure of the initial microbiota across multiple body habitats in newborns. Proc. Natl. Acad. Sci. USA107, 11971–11975 (2010). ArticlePubMedPubMed CentralGoogle Scholar
- Yatsunenko, T. et al. Human gut microbiome viewed across age and geography. Nature486, 222–227 (2012). ArticleCASPubMedPubMed CentralGoogle Scholar
- Le Chatelier, E. et al. Richness of human gut microbiome correlates with metabolic markers. Nature500, 541–546 (2013). ArticleCASPubMedGoogle Scholar
- Wesolowska-Andersen, A. et al. Choice of bacterial DNA extraction method from fecal material influences community structure as evaluated by metagenomic analysis. Microbiome2, 19 (2014). ArticlePubMedPubMed CentralGoogle Scholar
- McOrist, A.L., Jackson, M. & Bird, A.R. A comparison of five methods for extraction of bacterial DNA from human faecal samples. J. Microbiol. Methods50, 131–139 (2002). ArticleCASPubMedGoogle Scholar
- Smith, B., Li, N., Andersen, A.S., Slotved, H.C. & Krogfelt, K.A. Optimising bacterial DNA extraction from faecal samples: comparison of three methods. Open Microbiol. J.5, 14–17 (2011). ArticleCASPubMedPubMed CentralGoogle Scholar
- Maukonen, J., Simões, C. & Saarela, M. The currently used commercial DNA-extraction methods give different results of clostridial and actinobacterial populations derived from human fecal samples. FEMS Microbiol. Ecol.79, 697–708 (2012). ArticleCASPubMedGoogle Scholar
- Kennedy, N.A. et al. The impact of different DNA extraction kits and laboratories upon the assessment of human gut microbiota composition by 16S rRNA gene sequencing. PLoS One9, e88982 (2014). ArticlePubMedPubMed CentralGoogle Scholar
- Salonen, A. et al. Comparative analysis of fecal DNA extraction methods with phylogenetic microarray: effective recovery of bacterial and archaeal DNA using mechanical cell lysis. J. Microbiol. Methods81, 127–134 (2010). ArticleCASPubMedGoogle Scholar
- Ariefdjohan, M.W., Savaiano, D.A. & Nakatsu, C.H. Comparison of DNA extraction kits for PCR-DGGE analysis of human intestinal microbial communities from fecal specimens. Nutr. J.9, 23 (2010). ArticlePubMedPubMed CentralGoogle Scholar
- Sunagawa, S. et al. Metagenomic species profiling using universal phylogenetic marker genes. Nat. Methods10, 1196–1199 (2013). ArticleCASPubMedGoogle Scholar
- Manichanh, C., Borruel, N., Casellas, F. & Guarner, F. The gut microbiota in IBD. Nat. Rev. Gastroenterol. Hepatol.9, 599–608 (2012). ArticleCASPubMedGoogle Scholar
- Lozupone, C.A. et al. Meta-analyses of studies of the human microbiota. Genome Res.23, 1704–1714 (2013). ArticleCASPubMedPubMed CentralGoogle Scholar
- Raes, J. & Bork, P. Molecular eco-systems biology: towards an understanding of community function. Nat. Rev. Microbiol.6, 693–699 (2008). ArticleCASPubMedGoogle Scholar
- Voigt, A.Y. et al. Temporal and technical variability of human gut metagenomes. Genome Biol.16, 73 (2015). ArticlePubMedPubMed CentralGoogle Scholar
- Franzosa, E.A. et al. Relating the metatranscriptome and metagenome of the human gut. Proc. Natl. Acad. Sci. USA111, E2329–E2338 (2014). ArticleCASPubMedPubMed CentralGoogle Scholar
- Song, S.J. et al. Preservation methods differ in fecal microbiome stability, affecting suitability for field studies. mSystemshttps://dx.doi.org/10.1128/mSystems.00021-16 (2016).
- Gohl, D.M. et al. Systematic improvement of amplicon marker gene methods for increased accuracy in microbiome studies. Nat. Biotechnol.34, 942–949 (2016). ArticleCASPubMedGoogle Scholar
- Claassen, S. et al. A comparison of the efficiency of five different commercial DNA extraction kits for extraction of DNA from faecal samples. J. Microbiol. Methods94, 103–110 (2013). ArticleCASPubMedPubMed CentralGoogle Scholar
- Yuan, S., Cohen, D.B., Ravel, J., Abdo, Z. & Forney, L.J. Evaluation of methods for the extraction and purification of DNA from the human microbiome. PLoS One7, e33865 (2012). ArticleCASPubMedPubMed CentralGoogle Scholar
- Kultima, J.R. et al. MOCAT: a metagenomics assembly and gene prediction toolkit. PLoS One7, e47656 (2012). ArticlePubMedPubMed CentralGoogle Scholar
- Qin, J. et al. A human gut microbial gene catalogue established by metagenomic sequencing. Nature464, 59–65 (2010). ArticleCASPubMedPubMed CentralGoogle Scholar
- Huttenhower, C. et al. Human Microbiome Project Consortium. Structure, function and diversity of the healthy human microbiome. Nature486, 207–214 (2012). ArticleCASGoogle Scholar
- Franzosa, E.A. et al. Identifying personal microbiomes using metagenomic codes. Proc. Natl. Acad. Sci. USA112, E2930–E2938 (2015). ArticleCASPubMedPubMed CentralGoogle Scholar
- Powell, S. et al. eggNOG v3.0: orthologous groups covering 1133 organisms at 41 different taxonomic ranges. Nucleic Acids Res.40, D284–D289 (2012). ArticleCASPubMedGoogle Scholar
- Lozupone, C.A., Stombaugh, J.I., Gordon, J.I., Jansson, J.K. & Knight, R. Diversity, stability and resilience of the human gut microbiota. Nature489, 220–230 (2012). ArticleCASPubMedPubMed CentralGoogle Scholar
- Santiago, A. et al. Processing faecal samples: a step forward for standards in microbial community analysis. BMC Microbiol.14, 112 (2014). ArticlePubMedPubMed CentralGoogle Scholar
- InhibitEx Tablets - QIAGEN Online Shop. Available at: https://www.qiagen.com/fr/shop/lab-basics/buffers-and-reagents/inhibitex-tablets/.
- Henderson, G. et al. Effect of DNA extraction methods and sampling techniques on the apparent structure of cow and sheep rumen microbial communities. PLoS One8, e74787 (2013). ArticleCASPubMedPubMed CentralGoogle Scholar
- Jones, M.B. et al. Library preparation methodology can influence genomic and functional predictions in human microbiome research. Proc. Natl. Acad. Sci. USA112, 14024–14029 (2015). ArticleCASPubMedPubMed CentralGoogle Scholar
- Salter, S.J. et al. Reagent and laboratory contamination can critically impact sequence-based microbiome analyses. BMC Biol.12, 87 (2014). ArticlePubMedPubMed CentralGoogle Scholar
Acknowledgements
We thank S. Burz and K. Weizer for editing and web-posting the SOPs. We thank D. Ordonez and N.P. Gabrielli Lopez for advice on flow cytometry, which was provided by the Flow Cytometry Core Facility, EMBL. This study was funded by the European Community's Seventh Framework Programme via International Human Microbiome Standards (HEALTH-F4-2010-261376) grant. We also received support from Scottish Government Rural and Environmental Science and Analytical Services as well as from EMBL.
Author information
Authors and Affiliations
- Structural and Computational Biology, European Molecular Biology Laboratory, Heidelberg, Germany Paul I Costea, Georg Zeller, Shinichi Sunagawa, Melanie Tramontano, Marja Driessen, Rajna Hercog, Ferris-Elias Jung, Jens Roat Kultima, Matthew R Hayward, Luis Pedro Coelho, Kiran Raosaheb Patil & Peer Bork
- Department of Biology, Institute of Microbiology, ETH Zurich, Zurich, Switzerland Shinichi Sunagawa
- CEA - Institut François Jacob - Genoscope, Evry, France Eric Pelletier, Adriana Alberti, Laurie Bertrand & Céline Orvain
- CNRS UMR-8030, Evry, France Eric Pelletier
- Université Evry Val d'Essonne, Evry, France Eric Pelletier
- Metagenopolis, Institut National de la Recherche Agronomique, Jouy en Josas, France Florence Levenez, Michelle Daigneault, Philippe Langella, Emmanuelle Le Chatelier, Nicolas Pons, S Dusko Ehrlich & Joel Dore
- Department of Molecular and Cellular Biology, The University of Guelph, Guelph, Ontario, Canada., Emma Allen-Vercoe
- Department of Gastrointestinal Microbiology, German Institute of Human Nutrition Potsdam-Rehbruecke, Nuthetal, Germany Michael Blaut, Jana Junick, Delphine Saulnier & Kathleen Slezak
- School of Microbiology & APC Microbiome Institute, University College Cork, Cork, Ireland Jillian R M Brown & Paul W O'Toole
- Biofortis, Mérieux NutriSciences, Nantes, France Thomas Carton, Clémentine Mery & Milena Popova
- Danone Nutricia Research, Palaiseau, France Stéphanie Cools-Portier, Muriel Derrien, Anne Druesne, Johan van Hylckama Vlieg & Patrick Veiga
- Laboratory of Microbiology, Wageningen University & Research, Wageningen, the Netherlands Willem M de Vos, Hans Heilig & Erwin G Zoetendal
- Department of Bacteriology and Immunology, Immunobiology Research Program, University of Helsinki, Helsinki, Finland Willem M de Vos & Anne Salonen
- Michael Smith Laboratories, University of British Columbia, Vancouver, British Columbia, Canada B Brett Finlay
- Rowett Institute of Nutrition and Health, University of Aberdeen, Aberdeen, UK Harry J Flint, Jennifer C Martin & Karen P Scott
- Digestive System Research Unit, Vall d'Hebron Research Institute, Barcelona, Spain Francisco Guarner & Chaysavanh Manichanh
- Graduate School of Frontier Sciences, The University of Tokyo, Chiba, Japan Masahira Hattori
- Graduate School of Advanced Science and Engineering, Waseda University, Tokyo, Japan Masahira Hattori
- Texas Children's Hospital, Feigin Center, Houston, Texas, USA Ruth Ann Luna & James Versalovic
- Center for Medical Research, Medical University of Graz, Graz, Austria Ingeborg Klymiuk
- Department of Epidemiology, College of Public Health and Health Professions and College of Medicine, Emerging Pathogens Institute, University of Florida, Gainesville, Florida, USA Volker Mai
- Graduate School of Environmental and Life Science, Okayama University, Okayama, Japan Hidetoshi Morita
- Department of Medical Microbiology, School of Nutrition and Translational Research in Metabolism (NUTRIM) and Care and Public Health Research Institute (Caphri), Maastricht University Medical Center, Maastricht, the Netherlands John Penders
- Department of Bacteria, Unit of Foodborne Infections, Parasites & Fungi, Statens Serum Institut, Copenhagen, Denmark Søren Persson
- Department of Microbiology & Immunology and Robarts Research Institute, Centre for Human Immunology, University of Western Ontario, London, Ontario, Canada Bhagirath Singh
- Ministry of Education Key Laboratory for Systems Biomedicine, Shanghai Centre for Systems Biomedicine, Shanghai Jiao Tong University, Shanghai, PR China Liping Zhao
- King's College London, Centre for Host-Microbiome Interactions, Dental Institute Central Office, Guy's Hospital, London, UK S Dusko Ehrlich
- Department of Bioinformatics, Biocenter, University of Würzburg, Würzburg, Germany Peer Bork
- Molecular Medicine Partnership Unit, Heidelberg, Germany Peer Bork
- Max-Delbrück-Centre for Molecular Medicine, Berlin, Germany Peer Bork
- Paul I Costea